Alessandro Tengattini Habilitation à Diriger des Recherches (HDR)
Lundi 20 janvier 2025 13H00
Thermo-hydro-chemo-mechanical couplings in porous media through neutron and X-ray tomography and micro-inspired modelling.
This presentation will provide an overview of recent developments the combined use of neutron and X-ray imaging of coupled thermos-chemo-hydro-mechanical processes of porous media. This data is used to validate and calibrate micro-to-macro analytical and numerical models of these process.
Jury
- Jean-Michel PEREIRA (Professor) École Nationale des Ponts et Chaussées, Rapporteur
- Peter MOONEN (Professor) Université de Pau et Pays de l'Adour, Rapporteur
- Luc SALVO (Professor) Grenoble INP – UGA, Rapporteur
- Gilles PIJAUDIER-CABOT (Professor) Université de Pau et Pays de l'Adour, Examinateur
- Claudio DI PRISCO (Professor) Politecnico di Milano, Examinateur
- Gaël COMBE (Professor) Grenoble INP – UGA, Examinateur
- Nikolay KARDJILOV, Dr., Helmholtz Zentrum Berlin (HZB) Invité
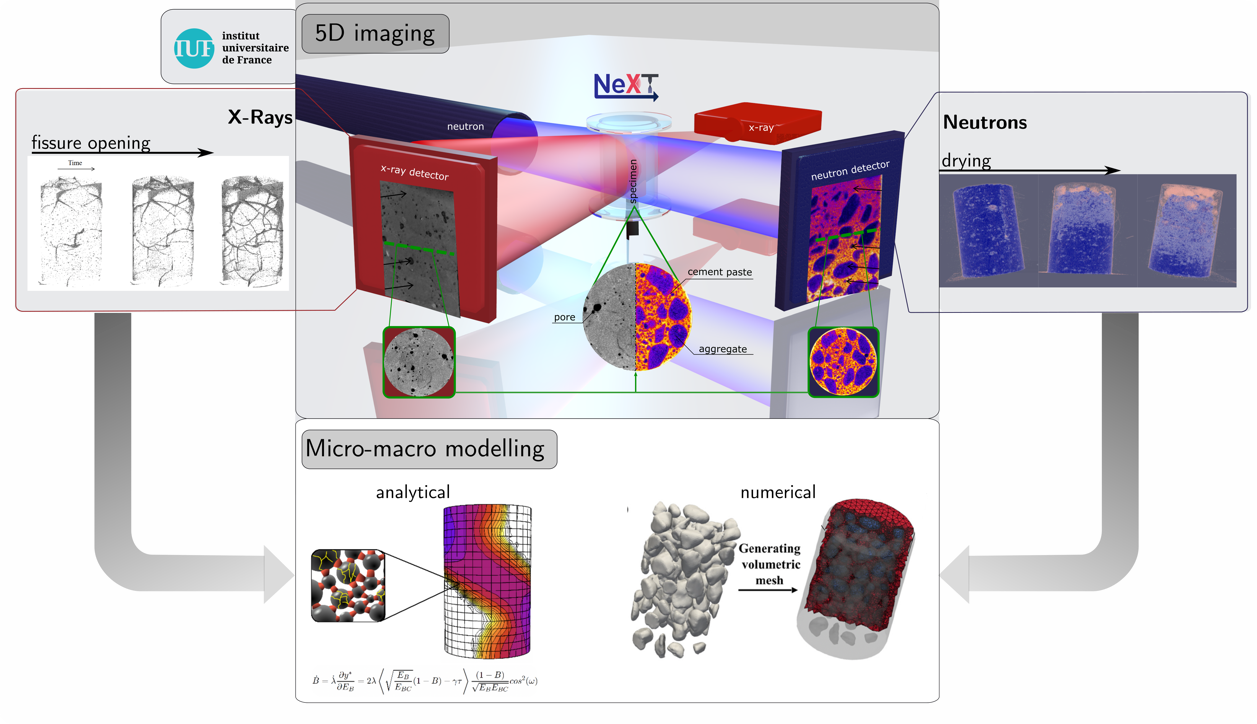
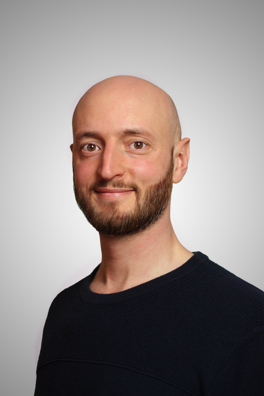
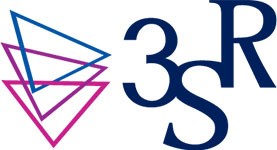
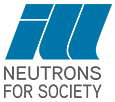
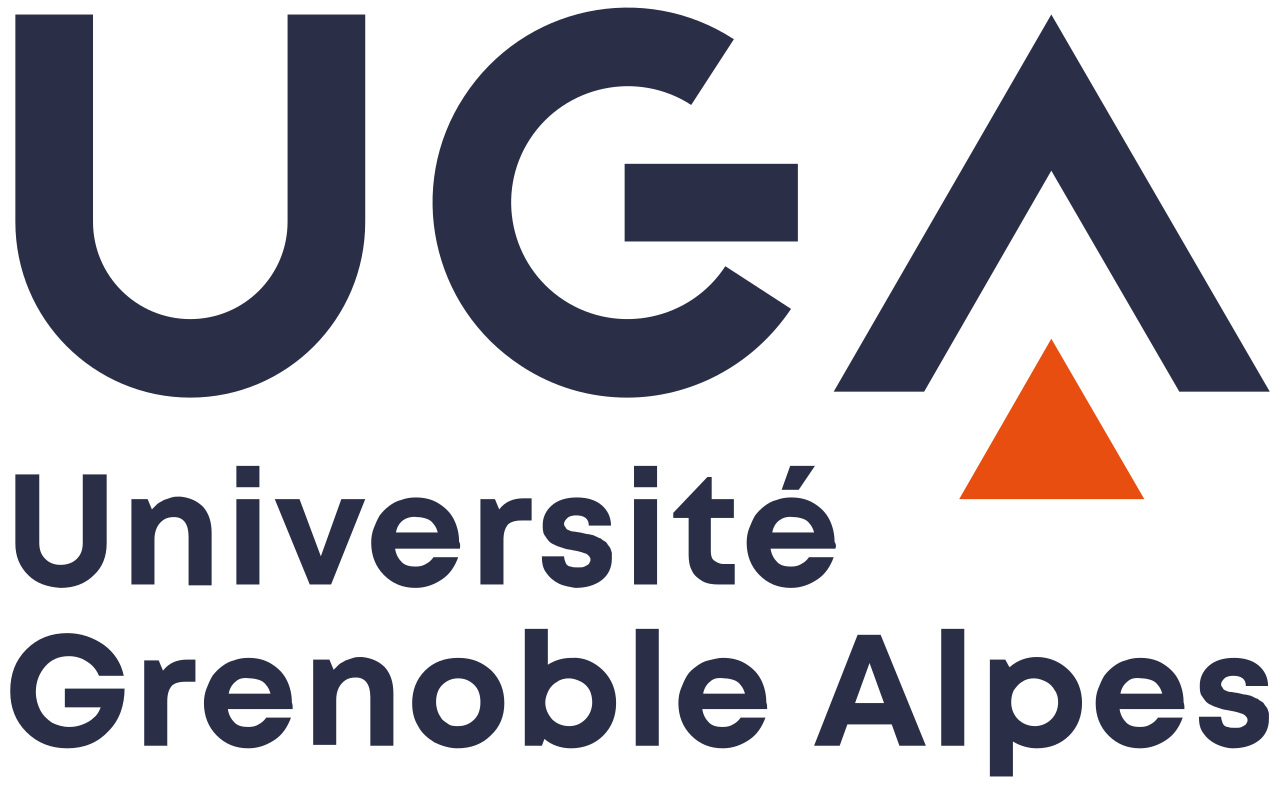